Science
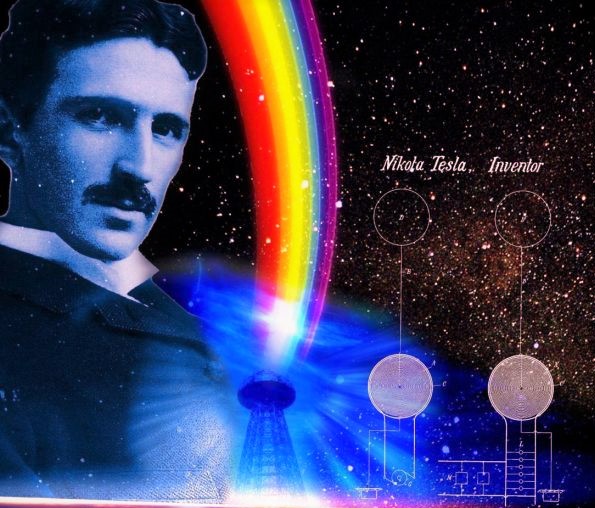
Science Problems
Academic Research Paywall
Paywall- The Business of ScholarshipVideosNews Articles Academic paywalls mean publish and perish - Al Jazeera Harvard University says it can't afford journal publishers' prices - Open access scientific publishing - The Guardian How much does it cost to get a...
Replication Crisis
VideosMainstream News - Replication Crisis 1,500 scientists lift the lid on reproducibility - Nature News & Comment A failed replication draws a scathing personal attack from a psychology professor - National Geographic Believe It Or Not, Most Published Research...
Scientific Research – Fraud and Misconduct
News Articles False positives: fraud and misconduct are threatening scientific research - The Guardian Fraud and Misconduct in Research - University of Michigan Press Fraud and the decline of science - The Guardian Misconduct behind most retractions of scientific...
Science Solutions
Free Academic Papers
Sci-Hub - removing barriers in the way of science Unpaywall- An open database of 20 million free scholarly articles
Open Science
VideosNews Articles Free Science Could Be the Answer to Our "Fake News" Epidemic - Futurism How to Access Paywalled Scientific Journal Articles - Life Hacker Publish and be praised - The Guardian Set science free from publishers' paywalls - New Scientist The Quest to...
Scientists
Nikola Tesla
Tesla - Master Of Lightning DocumentaryTesla VideosMainstream News Nikola Tesla Park- INNOVATION. POWER. PLACE. – Buffalo Rising Tesla - Master of Lightning- The Missing Papers - PBS Websites FBI Records- The Vault — Nikola Tesla Open Tesla Research - Inventions &...
Science
Electricity
VideosArchival FilmsShortsShock & Awe- The Story of Electricity - Spark (2011) 1-3Shock & Awe- The Story of Electricity - The Age of Invention (2011) 2-3Shock & Awe- The Story of Electricity - Revelations and Revolutions (2011) 3-3